Nonlinear Quantum Optics Group
Our group explores how we can create strong optical nonlinearity in a medium at light levels of individual photons. Such a quantum nonlinear medium mediates an effective interaction between single photons and thus opens the possibility of manipulating light at its most fundamental level. Our goal is to realize a system that enables full control over the quantum state of few photon light pulses.
Latest news
© ML4Q
Meet us at the Girls' Day 03.04.20251
Be a physicist for a day!
Join us on a journey into the world of quantum physics!
© nqo
Meet us at DPG2
We are excited for the upcoming DPG SAMOP spring meeting 2025 - that we are hosting in Bonn! ✨
Support services for employees and students3
Your well-being matters to us. We want to draw attention to the fact that the University of Bonn offers counselling and support services for employees and students.
Our research
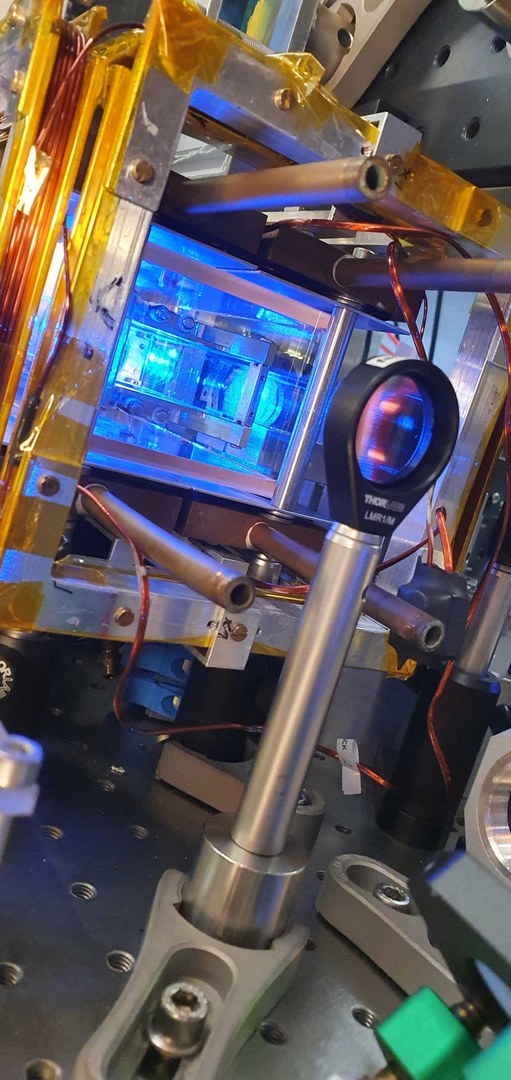
© nqo
Rubidium Rydberg Nonlinear Quantum Optics
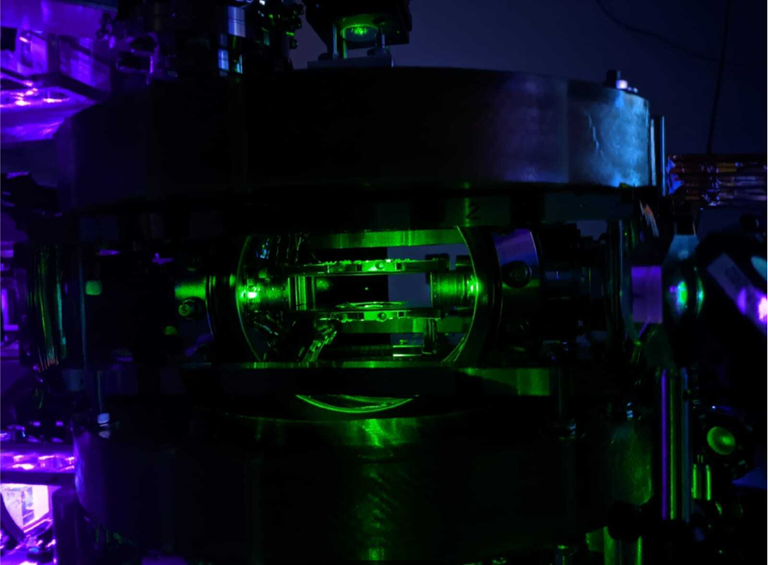
© nqo
Ytterbium Rydberg Nonlinear Quantum Optics

© nqo
Fiber Cavity Optomechanics
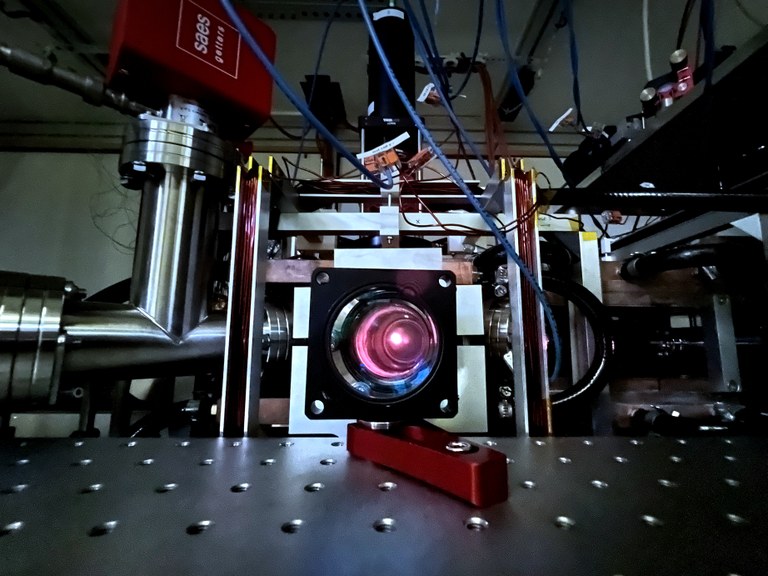
© nqo
Hybrid quantum optics
Get in touch!
Prof. Dr. Sebastian Hofferberth
hofferberth@iap.uni-bonn.de
Tel.: +49 (0)228 733477
Contact the institute
Institut für Angewandte Physik
Wegelerstraße 8
Universität Bonn
53115 Bonn, Germany
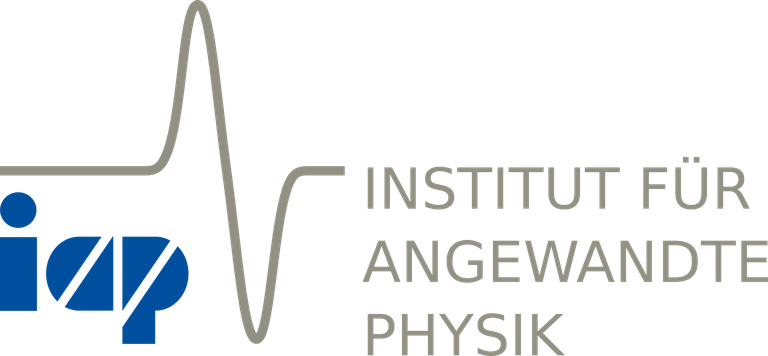
© IAP
Links
- https://www.iap.uni-bonn.de/nqo/en/nqonews/meet-us-at-the-girls-day-2025
- https://www.iap.uni-bonn.de/nqo/en/nqonews/meet-us-at-dpg
- https://www.iap.uni-bonn.de/nqo/en/nqonews/support-services-for-employees-and-students
- https://www.iap.uni-bonn.de/nqo/en/nqonews/rqo-paper-published-in-pra
- https://www.iap.uni-bonn.de/nqo/en/nqonews/latest-news
- https://www.iap.uni-bonn.de/nqo/en/research/rubidium-rydberg-nqo
- https://www.iap.uni-bonn.de/nqo/en/research/ytterbium-rydberg-nqo
- https://www.iap.uni-bonn.de/nqo/en/research/fiber-cavity-optomechanics
- https://www.iap.uni-bonn.de/nqo/en/research/hybrid-quantum-optics
- http://iap.uni-bonn.de