Nonlinear Quantum Optics Group
Our group explores how we can create strong optical nonlinearity in a medium at light levels of individual photons. Such a quantum nonlinear medium mediates an effective interaction between single photons and thus opens the possibility of manipulating light at its most fundamental level. Our goal is to realize a system that enables full control over the quantum state of few photon light pulses.
Latest news
Meet us at Pint of Science Bonn 20251
Join us on May 20th at Pint of Science in Bonn, where Theresa and Julia will introduce you to the fun world of Rydberg physics.
© nqo
Florian Giefer: New Master student2
Florian will stay with us as a Master student ✨
© nqo
New Bachelor students3
Teresa, Johnny, Sinja and Kaylan join the group as Bachelor students.
© ML4Q
Meet us at the Girls' Day 03.04.20254
Be a physicist for a day!
Join us on a journey into the world of quantum physics!
Our research
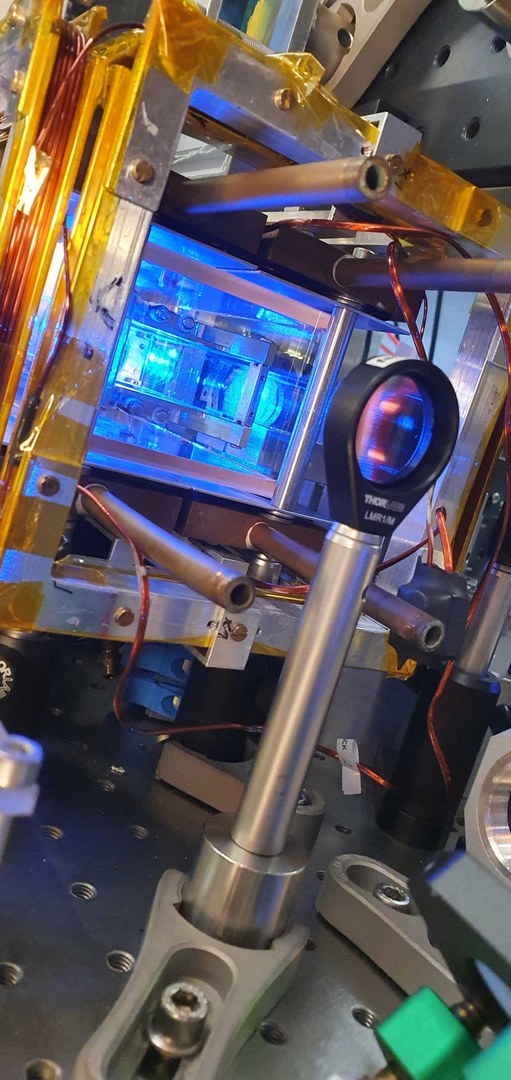
© nqo
Rubidium Rydberg Nonlinear Quantum Optics
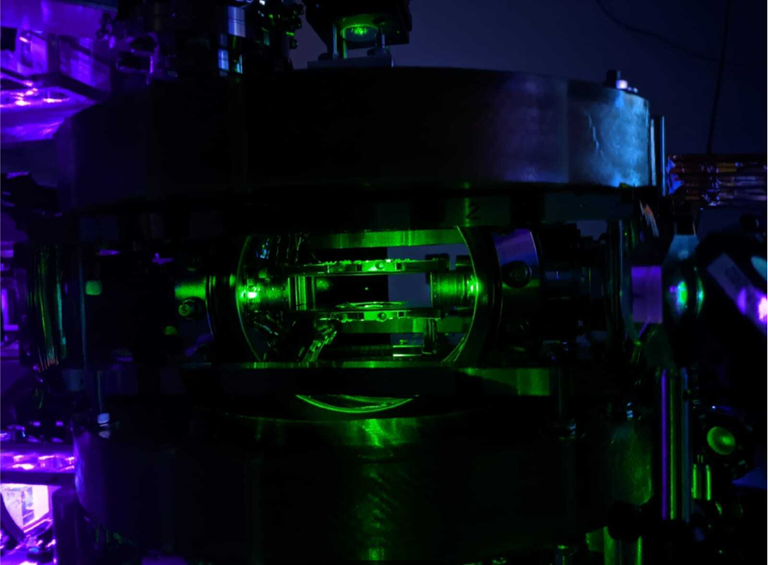
© nqo
Ytterbium Rydberg Nonlinear Quantum Optics

© nqo
Fiber Cavity Optomechanics
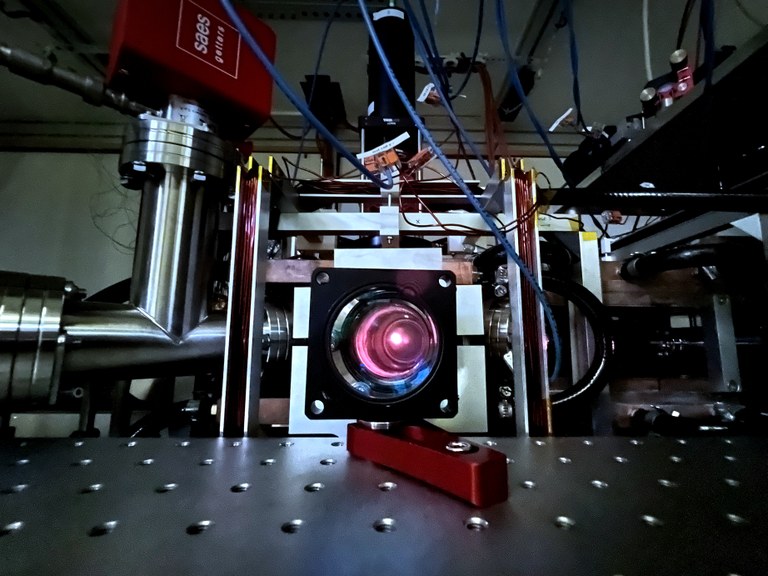
© nqo
Hybrid quantum optics
Get in touch!
Prof. Dr. Sebastian Hofferberth
hofferberth@iap.uni-bonn.de
Tel.: +49 (0)228 733477
Contact the institute
Institut für Angewandte Physik
Wegelerstraße 8
Universität Bonn
53115 Bonn, Germany
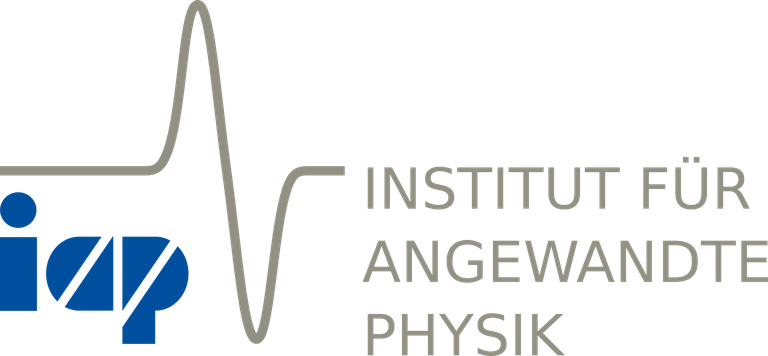
© IAP
Links
- https://www.iap.uni-bonn.de/nqo/en/nqonews/meet-us-at-pint-of-science-bonn-2025
- https://www.iap.uni-bonn.de/nqo/en/nqonews/florian-giefer-new-master-student
- https://www.iap.uni-bonn.de/nqo/en/nqonews/new-bachelor-students
- https://www.iap.uni-bonn.de/nqo/en/nqonews/meet-us-at-the-girls-day-2025
- https://www.iap.uni-bonn.de/nqo/en/nqonews/latest-news
- https://www.iap.uni-bonn.de/nqo/en/research/rubidium-rydberg-nqo
- https://www.iap.uni-bonn.de/nqo/en/research/ytterbium-rydberg-nqo
- https://www.iap.uni-bonn.de/nqo/en/research/fiber-cavity-optomechanics
- https://www.iap.uni-bonn.de/nqo/en/research/hybrid-quantum-optics
- http://iap.uni-bonn.de